Development of the mRNA vaccine
How basic research creates a launchpad for innovations
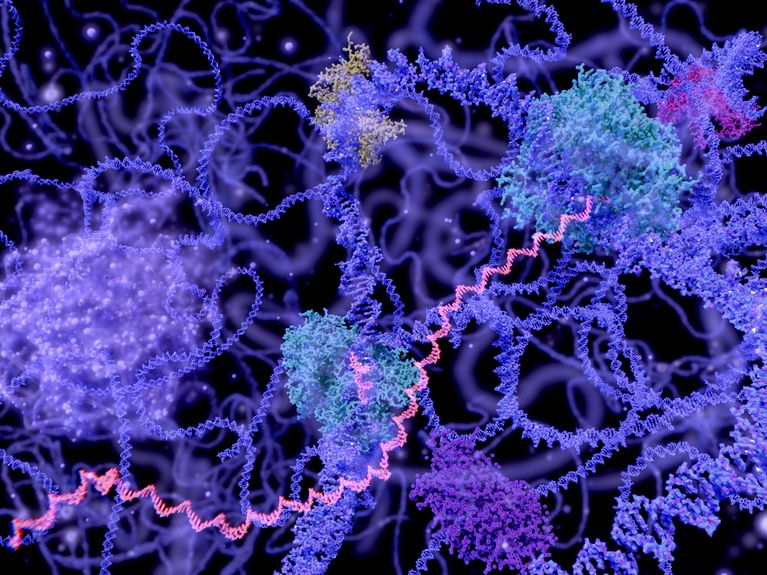
Picture: Juan Gaertner/Shutterstock.
The successful development of the mRNA active ingredient highlights the vital role basic research plays in applications.
The coronavirus pandemic triggered a real race among vaccine experts aiming to develop vaccines against SARS-Cov-2 infections. The first active ingredients were ready by spring 2020, just a few months after the initial outbreak of the disease. But one stood out as being particularly efficacious: This ingredient is based on messenger ribonucleic acid, or mRNA, which plays an important role in gene expression—the translation from DNA to protein. But how did researchers manage to achieve such staggering results in such a short space of time? How was this possible?
Heinrich Haas, an expert in physical chemistry and lead scientist at biotech firm BioNTech, considers this achievement to be much less surprising than it first appears. “The pharmaceutical development process for active ingredients to fight the pandemic put technologies into practice that the scientific community had already been working on for decades. mRNA is a molecule from the nucleotide family, which also includes deoxyribonucleic acid, or DNA, and other types of RNA such as small interfering RNA, known as siRNA. mRNA preparations offer numerous advantages compared to DNA preparations that researchers have already been looking at for some time.” For example, DNA preparations have to penetrate into the nucleus of the cell to become effective, and there are greater risks involved in using them as products for gene therapy. “The barriers are comparatively lower for mRNA therapy,” explains Haas. Even though the mRNA also needs to be incorporated by the cell to gain efficacy, it doesn’t need to penetrate into the nucleus. Besides this, mRNA is a safer pharmaceutical ingredient than DNA in and of itself because it cannot be integrated into the genetic material. “But because research in the area of DNA-based preparations had already been underway for some time, a lot of groundwork had already been laid that could now be applied in the development of mRNA medications, too. A great deal of the knowledge and methods developed in the course of DNA research went into our research on substances,” Haas adds.
Heinrich Haas. Bild: BioNTech
The research carried out by Ugur Sahin and Özlem Türeci, the founders of BioNTech, also has a lot of history behind it. Back at the end of the 1990s, the two scientists were already examining why the introduction of mRNA prompted a low immune response—a formidable question at the time. They were ultimately able to uncover the full potential of the RNA molecule thanks to a series of scientific and technological breakthroughs.
As with conventional vaccines, the special mRNA vaccine relies on training the body’s immune system to fight the SARS-Cov-2 pathogen. The vaccine’s mRNA contains the construction manual for the spike protein fragment from the shell of the coronavirus. After being absorbed into the cells, the spike protein is synthesized by the cells and presented to the immune system. The immune system responds, and this response equips it to defend the body against the actual coronavirus.
Even though the mRNA—in contrast to DNA—doesn’t need to penetrate into the nucleus in order to achieve its therapeutic effect, it still has to be first incorporated into the cell and thus pass through the cell membrane. “The cell membrane is a very efficient barrier in terms of keeping undesirable substances from getting in. The mRNA is a very large molecule with a negative charge that is repelled by the cell membrane and therefore isn’t immediately absorbed by the cell,” Haas notes. In order to overcome this barrier, he explains that the mRNA has to be packaged in suitable nanoparticles. These nanoparticles can consist of so-called lipids, or fat molecules, similar to those found in the natural cell membrane. Haas says, “Lipids have been the subject of research and development in the pharmaceutical sector for decades and are already being used in clinical practice in the form of liposomes, for example. Lipid-based nanoparticles are a preferred means of transport in the development of therapy approaches based on nucleotides like DNA and RNA as well.” In recent years, this approach has also yielded lipid nanoparticles (LNP) that are used in mRNA therapeutics. “The mRNA molecules are embedded in LNPs to ensure they aren’t immediately degraded by enzymes after being injected and are instead transported to the target cells and absorbed there. The cells can then synthesize the spike proteins, and this triggers the first step toward an immune response,” Haas says.
Analyzing the behavior of the molecules as quickly and effectively as possible is a key aspect for research. In this case, the relevant analyses were made possible by collaboration using PETRA III, the large-scale accelerator-based research facility at DESY. This facility provided a superb means of characterizing the behavior of such lipid nanoparticles in detail. The high-intensity, extremely focused X-ray of the 2.3-kilometer ring accelerator was used to examine the mRNA nanoparticles used by BioNTech. “We used a measuring method known as small-angle X-ray scattering,” explains Dmitri Svergun of the European Molecular Biology Laboratory (EMBL), who oversees EMBL’s P12 beamline at DESY in Hamburg. These types of measurements are taken by firing PETRA III’s X-ray at the biological molecules in an aqueous solution; that is, essentially in their natural environment. The radiation is scattered very slightly during this process. “The type of scattering lets us draw conclusions about the structures of the corresponding samples.” Svergun sees synchrotron radiation facilities like PETRA III as ideally suitable for this type of analysis. “The X-ray has to be extremely focused and powerful because we’re looking for the rays that are not scattered on the sample, or are only scattered to a minimal extent,” Svergun says. “These slightly diffracted rays are particularly effective in helping us pinpoint the entire structure of particles.”
For Svergun and Haas, the collaboration that has gone into researching the vaccine is a prime example of how basic and applied research can be combined successfully. “If you look at the impact that the results of this work can have in terms of fighting the pandemic,” Svergun says proudly, “this longstanding collaboration between EMBL at DESY, BioNTech, the University of Mainz, and other partners has really paid off.” Haas, too, is impressed by the research and results it has yielded. But he thinks it is still far from achieving its maximum impact; in fact: “I think this research work can make a key contribution to the development of new mRNA drugs. We’re right at the beginning of a paradigm shift. mRNA gives us a completely new class of substances that are capable of almost everything that the existing conventional protein substances can do, but can do it much better and more efficiently.” Haas and BioNTech are working not just on mRNA therapies against coronavirus but also on mRNA-based cancer treatments. He sees in-depth knowledge from basic research as essential to these efforts, along with extensive collaboration.
[Translate to Englisch:]
[Translate to Englisch:]
Für Dmitri Svergun und Heinrich Haas ist die Zusammenarbeit im Rahmen der Impfstoff-Forschung ein Paradebeispiel für erfolgreiches Zusammenspiel von Grundlagenforschung und angewandter Forschung. „Wenn man in Betracht zieht, welchen Impact das Ergebnis solcher Arbeiten bei der Bekämpfung der Pandemie haben können“, sagt Svergun stolz, „hat sich diese langjährige Kollaboration, bestehend aus EMBL am DESY, BioNTech, der Universität Mainz und anderen wirklich gelohnt.“ Auch Heinrich Haas ist von der Forschung und ihren Ergebnissen begeistert. Für ihn ist das Maximum aber noch lange nicht erreicht, im Gegenteil: „Ich denke, diese Forschungsarbeiten können einen wesentlichen Beitrag für die Entwicklung neuer mRNA Medikamente leisten. Wir stehen am Anfang eines Paradigmenwechsels. Mit der mRNA erhalten wir eine vollkommen neue Klasse von Wirkstoffen, die fast alles können, was auch die bisherigen konventionellen Protein-Wirkstoffe tun, aber viel effizienter und besser.“ Heinrich Haas und BioNTech arbeiten nicht nur an mRNA-Therapien gegen das Corona-Virus, sondern auch an mRNA-basierter Krebstherapie. Dafür ist aus seiner Sicht ein vertieftes Wissen durch Grundlagenforschung unerlässlich. Und viel Zusammenarbeit.
Simone Spuler. Picture: Pablo Castagnola
Simone Spuler, lead scientist at the Max Delbrück Center (MDC) and an expert in congenital muscle disorders, says basic research on mRNA is also opening up new possibilities in her field of research: “Here at the MDC, we’re working on so-called muscular dystrophies.” These are genetic diseases that lead to muscle loss, and according to Spuler, there are around 50 different diseases—and thus 50 different genes—that can result in fatal muscle wasting due to mutations. “The discovery of the CRISPR/Cas9 gene scissors opened up completely new prospects for us in terms of curing this disease, which was previously considered untreatable.” CRISPR/Cas9 cuts the genome of a living cell at a designated location, removes the mutated genes, and replaces them with new ones. Before this can happen, the gene scissors have to be introduced into the corresponding cell. The initial intention was to use ring-shaped DNA structures for this task. But in the laboratory, it quickly became clear that ring-shaped DNA isn’t suitable for therapy on a patient. “This is because DNA structures as small as these aren’t very mobile; they are either quickly degraded or can be integrated into the genome,” Spuler explains. “In the case of the latter, we would have been placing gene scissors in the genome for the rest of the person’s lifetime, and this isn’t desirable.”
Spuler’s group instead looked for a different molecule that would be short-lived but could also function as gene scissors. “And that’s when we came up with the idea involving mRNA,” she says. “It can be used to make the gene scissors but is broken down in the cell after a day or two. This means the gene scissors, which have completed their repair work in the meantime, are no longer there.” Spuler considers this to be a very safe concept that researchers will be able to transfer to clinical application step by step thanks to the outstanding scientific results achieved on muscle stem cells. This work will present significant challenges for Spuler’s team as they move forward with their research. For one, the mRNA has to be packaged for transportation into the body, as is already occurring in practice with the COVID-19 vaccines. Besides this, it has to arrive at the relevant muscle before it passes through the muscle membrane. “Packaging and sending it off to the right recipient so it can then be absorbed in the right muscle cell and incorporating all these aspects into a therapy that reaches all of the diseased muscles—it’s really very challenging,” Spuler acknowledges. At the same time, this approach has already proven successful in the laboratory. The mRNA-based gene scissors work. Spuler’s research group will now be taking the next key step in fighting muscular dystrophy soon—transferring the results and concepts from basic research to a clinical study. “The study is designed such that we will be injecting muscle stem cells that we can already successfully propagate and repair in the lab into patients’ individual muscles. We are only conducting this clinical study to see whether new muscle cells can be built up to start with, but the patient won’t benefit much from them in the clinical sense. It goes without saying that this presents an ethical problem for us. Because we are giving patients hope of being cured, but we won’t be able to make these hopes a reality yet in this study.”
Spuler understands that basic research takes time. “But when we talk about a therapy plan and want to work on implementing it, we have to start by taking these small steps.” And Spuler is convinced that at some point, these many small steps will add up to a larger leap forward. She feels confident about her work: “I’m hopeful that I will be able to see a breakthrough in the treatments we can use to fight this insidious disease before the end of my career.”
Both of these examples underscore the ongoing and intensive preparatory work that has to go into basic research over many years and decades before concrete and sometimes astonishing applications suddenly come to light. Interdisciplinary interaction between different research fields—the same approach Hermann von Helmholtz already put into practice over 150 years ago—can be crucial to achieving this. For example, the synchrotron radiation Dmitri Svergun mentioned, which occurs during the acceleration of electrons, was discovered almost 75 years ago to the day, though it was initially only seen as a disruptive energy loss from the accelerated particles. Today, facilities like PETRA III are proving to be unimaginably beneficial light sources—including in the field of medicine and mRNA research.
Readers comments