Quantum Year 2025
Part #04: Neutrinos – Putting chameleons on the scales
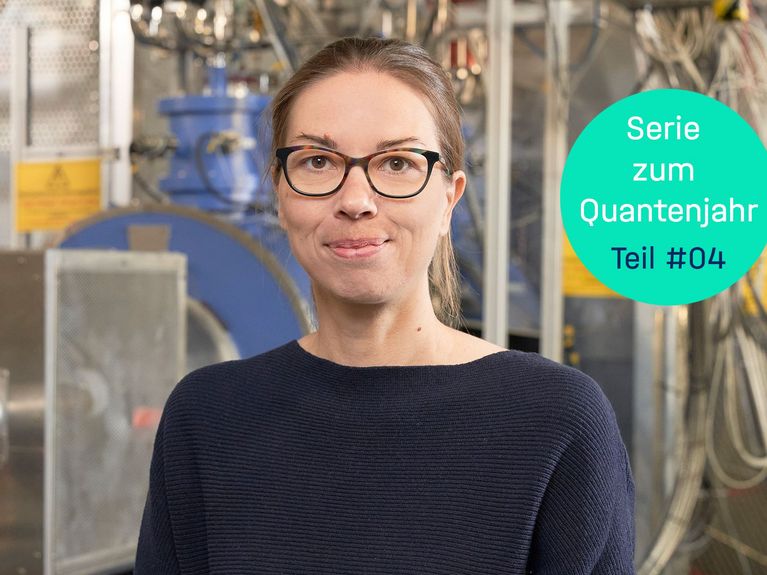
They’re super-light and fly through the universe – and through us – en masse, yet are almost impossible to grasp: in our fourth instalment for the Quantum Year, we tag along with Kathrin Valerius from the Karlsruhe Institute of Technology (KIT) on the trail of the elusive neutrinos.
100 years ago, when physics reinvented itself with the advent of quantum mechanics, a whole new world opened up for scientists. First, the wave-particle duality, the Schrödinger equation and Heisenberg’s uncertainty principle, used to describe the physics of atoms. Then, with the development of the Dirac equation incorporating the special theory of relativity, the prediction of antimatter, which is sometimes easy to create but doesn’t survive long in our material world. And the advances continued, one after the other: in experiments, the known particles such as electrons, protons and neutrons were joined by other, previously unknown ones – a veritable menagerie of particles. Among them was an inconspicuous type that our universe is teeming with: neutrinos.
“The neutrino is the second most common particle in the universe after the photon, the carrier of light,” explains Kathrin Valerius from KIT. On average, there are around 330 neutrinos in every cubic centimeter of the universe. Our sun alone produces more than 1039 neutrinos per second, some of which reach our Earth roughly eight minutes later. “In turn, one hundred billion of them pass through the tip of our index finger every second.” An incomprehensibly large number, so why don’t we notice anything? “Because they are electrically neutral and only interact weakly with other elementary particles, and therefore hardly interact with us at all,” says Valerius. It’s hardly surprising that it took almost twenty-five years for their existence to be experimentally proven in the mid-1950s, after being theoretically predicted by Wolfgang Pauli and Enrico Fermi.
“Today we know: there are three types of neutrinos,” says Valerius, “But for a long time it was unclear whether they had mass or not.” We now know that, too. And this is linked to the solution of a fundamental puzzle that had dominated astroparticle physics until recently: the solar neutrino problem. “The neutrinos produced in the sun’s nuclear fusion processes, which were measured in our detectors after their journey through space, did not match the expected values from the solar model.” Only later did scientists discover that the neutrinos of any given type of particle can transform into either of the other two types on their way from the sun to Earth, and that they oscillate quantum mechanically between states. “However, if all three types of solar neutrinos are measured, there is very good agreement between the measured neutrino flux and the model prediction,” explains Valerius. This neutrino oscillation can be explained quantum mechanically. “But only”, Valerius stresses, “if the neutrinos have a mass. They are the chameleons in the standard model.” And absolute lightweights. Because if they have any mass at all, it must be infinitesimally small. Nevertheless, according to Valerius, they play a decisive role in the universe, as they are “cosmic architects” and helped shape the formation of the large cosmic structures that we can see today.
But how much mass do neutrinos have? “To find out, we built the Karlsruhe Tritium Neutrino Experiment, KATRIN for short.” The large-scale research facility at KIT, which is operated in an international collaboration, comprises an ultra-pure gaseous tritium source and a huge mass spectrometer with a diameter of 10 meters over a total length of around 70 meters. “KATRIN makes it possible for the first time to determine direct upper limits in the sub-electron volt range,” says Valerius. “And these measurements don’t require any model assumptions about the nature of neutrinos or about the components of the standard cosmological model and so on.” KATRIN uses the latest quantum technologies for its sensors. Since KATRIN at the latest, it has been clear that neutrinos have unimaginably small masses compared to other elementary particles. “Thanks to the latest results from KATRIN, we now know that neutrinos are at least a million times lighter than electrons.” Though extremely light by particle physics standards, their tiny mass is crucial to understanding fundamental physical processes in the universe.
Neutrinos may therefore also represent one of the biggest symmetry breaks of all time. For shortly after the Big Bang, there must have been a tiny surplus of matter in the ultra-hot primordial universe of matter and antimatter that was able to escape being annihilated by antimatter – which now makes up all observable matter in our universe, including us humans. But what was responsible for this tiny imbalance between matter and antimatter in the first seconds of the universe’s existence? “We believe that neutrinos could have played a significant role here. For example, if they were identical to their antiparticles, thereby enabling particle physics processes that violate certain quantum numbers.”
Could neutrinos also be behind the mystery of dark matter? “Dark matter makes up over 80 percent of all matter in the universe. In other words, almost a quarter of the total energy density of the cosmos. And so far, we have no clear indication of what this dark matter is made of,” says Valerius. “The neutrinos we know of so far share particle properties that we attribute to dark matter.” However, they are too light to be the main component. “But hypothetical super-heavy neutrinos, or what we call ‘sterile neutrinos,’ could be a possible candidate to explain dark matter.” Which is why one aim of the upcoming second measurement phase of the KATRIN experiment will be to search for these sterile neutrinos. “With the planned upgrade of the KATRIN detector, we will seek to answer the question: are there more than three types of neutrinos? And do we find indications of physics beyond the standard model in the KATRIN measurements, for example new types of interaction?” After quantum mechanics, quantum field theory and quantum control (see our Quantum Year instalments #01, #02 and #03), a clue to this would undoubtedly be the beginning of another great quantum revolution after these first hundred years of quantum physics. Stay tuned!
Readers comments