Serie in the Quantum Year 2025
Part #03: The challenge of quantum computing or the art of juggling ping pong balls with mittens on
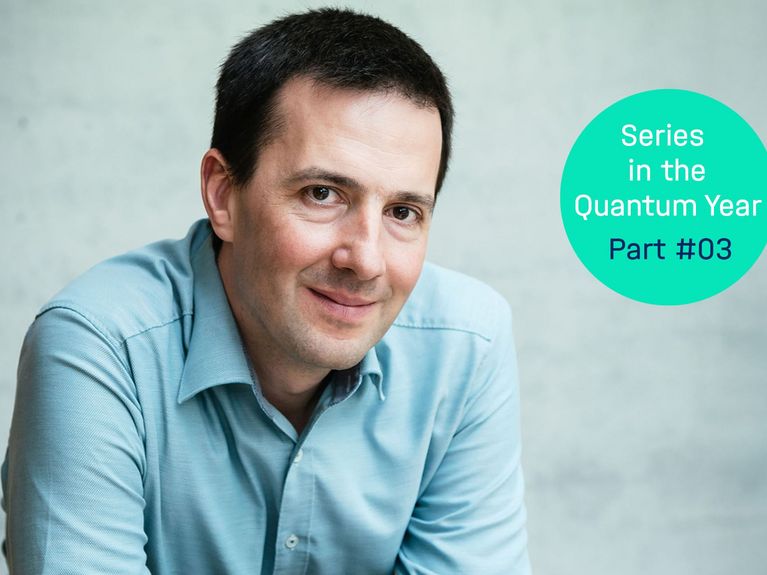
In the future, quantum computers might be able to complete tasks that conventional computers simply can’t. Ioan Pop, a quantum physicist and professor at the Karlsruhe Institute of Technology (KIT), explains how quantum computers work and the hurdles that need to be overcome in their design and operation.
Although our classical ideas of the states in the microcosm ultimately failed, Heisenberg and Schrödinger managed to formally describe these states within the framework of their quantum mechanics. For decades, research was limited to the indirect measurement of such quantum states. But today these quantum states can be coherently observed and manipulated without being destroyed, e.g. using quantum computers. But what does that term mean, and how do they differ from conventional computers?
“In conventional computers, digital data processing is based on binary numbers, which are represented by stringing together the smallest units of information, the binary digits (bits for short),” explains KIT quantum physicist Ioan Pop. “These bits only have two potential states: ‘zero’ or ‘one’.” Once logically interconnected, calculations based on instructions – algorithms – become possible. In principle, a quantum computer works in a similar way. However, in contrast to the bits used by a conventional computer, a quantum computer uses so-called quantum bits (qubits) as the smallest unit of information. “This allows the quantum mechanical superposition of the states ‘zero’ and ‘one’.” For Pop, this is comparable to tossing a coin in the air, but with the two potential states of the coin (heads up and tails down or heads down and tails up) superimposed, as if the coin remained suspended, perpetually spinning in midair. In quantum mechanics, this state is referred to as superposition. In a quantum computer, however, qubits can not only be superimposed but also entangled with each other – as if several perpetually spinning coins were thrown into the air and merged together. “With four bits, exactly one natural number between 0 and 15 can be represented in a computer. With four qubits, on the other hand, all 16 numbers can be represented simultaneously.”
For Pop, the major advantage of quantum computing over conventional computer systems is therefore the dramatic reduction in computing times in the case of special algorithms. As an example, he cites the “Shor” algorithm, which can be used to determine the divisors of composite numbers. “This algorithm plays a prominent role in encryption for modern electronic communications.” When wielded by a quantum computer, it is virtually a universal key in the context of cryptography. The international interest in its practical applications is correspondingly high. However, according to Pop, quantum computing also offers wholly new opportunities for basic research, e.g. in the modeling of fundamental chemical processes at the quantum level.
Despite the exciting applications, in Pop’s view it is the enduring fascination with the quantum mechanics of Heisenberg and Schrödinger that attracts the majority of researchers to quantum computing. “This pure curiosity about how to create and manipulate quantum mechanical systems and to achieve results that aren’t remotely intuitive,” he says enthusiastically. “What exactly is happening? How does nature work? What’s going on behind the quantum mechanical curtain?”
A quantum mechanical system can only maintain coherence as long as this “magical curtain” remains closed. Even the slightest external disturbance, the slightest observation of the system, causes the superposition of quantum states to collapse, resulting in what is known as decoherence, where the quantum mechanical system becomes a classical one. “This is also the greatest technical hurdle in quantum computing – maintaining quantum coherence.” Accordingly, in his view it’s not actually the often-invoked number of qubits in a quantum computer that is decisive as an indicator of success, but rather how long decoherence can be prevented. “The challenge is to link and control the qubits in such a way that they remain coherent for a sufficiently long time and allow reliable operations.” Like juggling ping pong balls with mittens on.
“There are different approaches to producing qubits.” Pop and his working group at KIT are concentrating on superconducting quantum circuits. In this approach, each qubit consists of an arrangement of certain atoms that behave similarly to a single atom at very low temperatures. For a functioning and high-quality quantum computer system, the delicate qubit must be integrated into the hardware of a computer system. It is therefore necessary to isolate the quantum states from the natural environment as effectively as possible, while also subjecting them to classical processes – to pet Schrödinger’s cat, so to speak, without knowing whether it’s alive or dead.
“The first prototypes with a small number of qubits are already available,” explains Pop, not without a measure of pride. “We’re working closely with our colleagues at the Forschungszentrum Jülich, who are focusing on a similar approach using superconducting quantum circuits.” Due to the complexity of quantum computing, it is crucial that interdisciplinary expertise comes together – for example, in the German project Qsolid, which involves a total of 26 partners from research and industry alongside the Forschungszentrum Jülich and KIT, and for which Ioan Pop is coordinating KIT’s activities. The first prototype, with ten qubits, went into operation in late 2024. In the coming years, the system is to be further expanded and integrated into Jülich’s existing supercomputer environment, for use in academic and industrial research alike.
One thing is for sure: the quanta will continue to surprise us – stay tuned for the next installment in this series!
Infographic:
Infographic: Franziska Roeder
Readers comments